
Features
Research
Technology
A precision oxygen control system for RAS: Freshwater Institute
April 22, 2024 By Scott Tsukuda and Steve Summerfelt
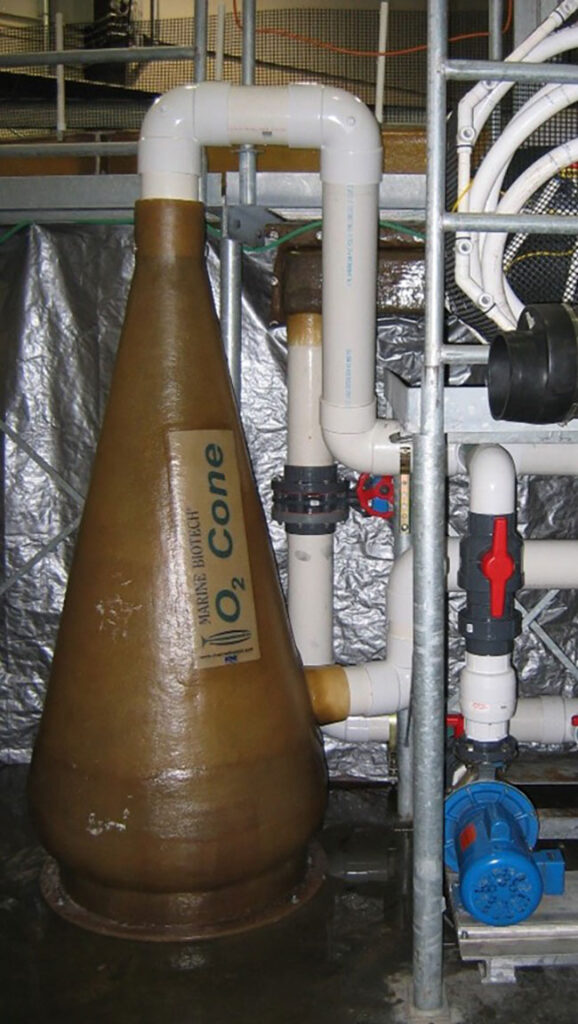
Controlling dissolved oxygen using pure oxygen is critical in intensive RAS systems. Published literature indicates that oxygen use can be three to five per cent of overall production costs. As with feed or electricity inputs, it is important to manage this resource efficiently. Yet, oxygen is often managed by setting gas use at fixed levels and then adjusting gas flow based on operator-observed dissolved oxygen (DO) concentration levels and know-how.
RAS systems are dynamic, and oxygen demand changes around planned events such as feeding or harvest or when unplanned events occur, such as equipment failure, power outages, or disease outbreaks.
Operators may manually set a higher-than-needed DO level by increasing oxygen gas flow to account for normal variation throughout the day while relying on a backup oxygenation system with fine bubble diffusers to activate when concentrations drop below a pre-determined level to ensure fish have adequate DO.
This approach is safe because unlike dissolved carbon dioxide or supersaturated nitrogen, higher oxygen levels are not typically a fish health concern. However, maintaining higher-than-needed DO levels is inefficient and can be improved by controlling DO based on real-time data.
Precision oxygen control
This study investigated using a side-stream oxygenation system to provide supplemental oxygenation on demand in a 150 m3 tank growing rainbow trout (Oncorhynchus mykiss).
The side-stream oxygenation system was comprised of a 3-HP centrifugal pump that pulled a side-stream flow of 1,100 L/min (290 gpm) from the fish culture tank and delivered it through a Downflow Bubble Contactor (DBC) operated at approximately 0.7 bar.
The side-stream flow was oxygenated in the DBC and then combined with normally oxygenated water in the primary RAS return flow just before entering the fish culture tank. DO measured at the fish culture tank sidebox provided the data to a PLC that controlled an actuated needle valve to vary the amount of oxygen gas injected at the DBC.
The DBC increased the DO in the side-stream flow, providing sufficient supplemental oxygen for the fish while also reducing the variability of the DO concentrations in the tank. Most of the oxygen demand of the fish was met by oxygen addition at the RAS low head oxygenation unit (LHO). The combination of DBC and LHO oxygenation allowed a target DO level in the fish culture tank to be maintained at all times, e.g., a setpoint of approximately 110% of dissolved oxygen saturation. Oxygen concentrations were measured at the tank dual-drain sidebox.
DBC oxygenation works by increasing the residence time of oxygen gas in the water column at a pressure of approximately 0.7 bar. The residence time of oxygen gas increases because as water flows down through the cone the cross-sectional area increases and the water velocity slows. The water velocity decreases to the point that it equals the rise velocity of the injected oxygen gas bubbles; at that point, oxygen bubbles are held in position until they dissolve into the water.
DBCs are expected to achieve 80-90% oxygen absorption efficiency at oxygen gas injection rates of 0.5% of the water flow rate. This is slightly better than the 70-90% oxygen transfer efficiency at the LHO and significantly better than the 3-10% oxygen absorption efficiency of fine bubble diffusers expected in our fish culture tank.
- Figure 1. Process flow diagram showing the side-stream loop where water was pumped from the fish culture tank, oxygenated in the downflow bubble contractor (DBC) and then returned just before the primary inlet.
- Figure 3. Comparison of fish tank DO concentration between no PLC control (static) and PLC controlled oxygen addition.
Observations
During the course of the study, the combined oxygen gas flow rate to the LHO and DBC varied between 2.3 and 50.4 L/min. The oxygen injected at the DBC alone ranged between 0 and 17.5 L/min. On a daily average basis, the oxygen gas injected at the DBC was 0-66% of the total oxygen gas added to the system. Daily oxygen addition peaked at 67.5 kg/day and decreased to 13.0 kg/day as fish were harvested and the tank biomass reduced from 8,450 kg to 3,400 kg.
Dissolved oxygen dips occurred numerous times each day when operating without oxygen control using the DBC. Supplemental oxygen addition at the DBC using Proportional-Integral (PI) control was found to maintain fish tank DO concentrations within acceptable levels over a variety of RAS operating conditions. Once fully implemented, the automatic DO control using the side-stream DBC maintained the target DO of 11 mg/L in the culture tank at all times.
System DO concentrations were observed during a harvest event. Harvest events require withholding fish feed for 24 hours or more before the event. Once feeding was stopped, oxygen consumption in the tank decreased. The PI control system responded by reducing and then stopping the addition of oxygen gas at the DBC.
During the study it was observed that the DO provided at the LHO could be more than needed by the fish, and while no oxygen was supplied via the DBC, some oxygen gas was wasted at the LHO. This wastage could be eliminated by lowering the baseline levels of DO supplied by the LHO, and allowing the control system to manage the oxygen addition using the DBC.
Oxygen efficiency and cost savings
Before starting the study, the tank DO concentration averaged 10.7 mg/L (S.D. 0.38 mg/L) when using the LHO alone to control DO in the fish tank. After the implementation of the side-stream DBC supplemental oxygen system, the tank DO concentration averaged 11.1 mg/L (S.D. 0.22 mg/L).
Between 20 and 60 per cent of oxygen was estimated to be saved each day with the side-stream control strategy while maintaining a higher average dissolved oxygen concentration.
Potential applications
This approach to controlling oxygen could be used in new RAS designs and as a retrofit to existing RAS to improve oxygenation efficiency and decrease operating costs.
At the Freshwater Institute, we have studied maintaining swimming speeds of at least 1 body length per second for post-smolt Atlantic salmon (Salmo salar) to improve growth rate and fish health and welfare. Maintaining water velocities for large salmon typically requires the use of a pumped, side-stream velocity assist system.
If a pumped velocity assist system is utilized, a DBC could be integrated and allow optimization of both tank water velocity and oxygenation. This approach would save energy as both the supplemental oxygen and velocity assist would not need to be operated until the biomass and fish required it.
Finally, this type of side-stream oxygenation system could be shared by a group of tanks that have different age fish cohorts, all in a single RAS module. Mounting the side-stream equipment to a moveable pallet or cart would allow the system to be moved from tank to tank as the biomass increases in one tank and is low in others. This further increases the cost efficiency and utility of the equipment.
This work was supported by the United States Department of Agriculture, Agricultural Research Service (USDA-ARS) under grant agreement number 59-1930-1-130.
Scott Tsukuda is director of operations at The Conservation Fund’s Freshwater Institute in Shepherdstown, W. Va. (stsukuda@conservationfund.org)
Steve Summerfelt, PhD is chief science officer of Superior Fresh in Hixton, Wis. (steve@superiorfresh.com)
Print this page